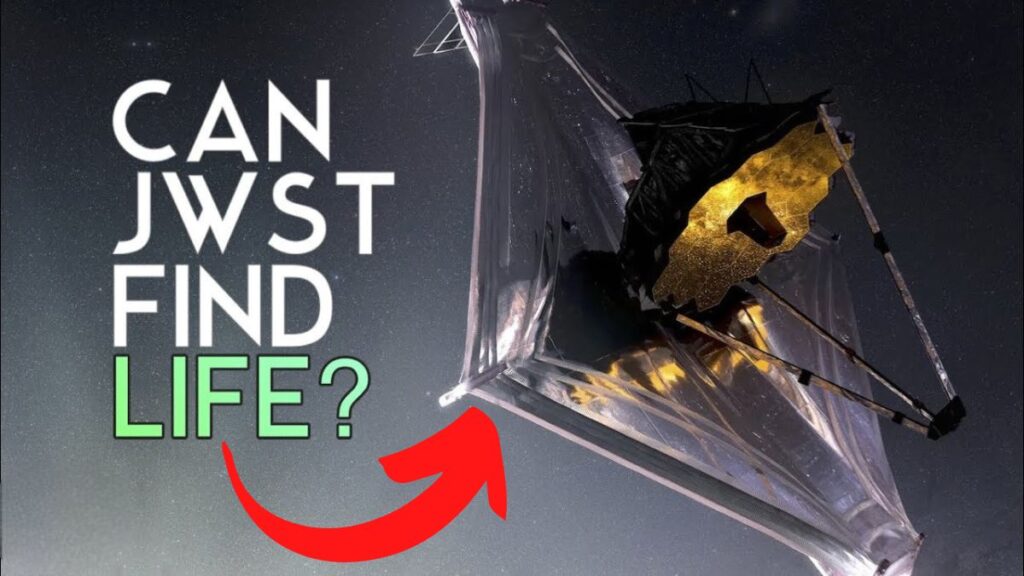
JWST is humanity’s premier astronomical telescope.
It represents the pinnacle of our engineering capabilities as well as our scientific aspirations.
It’s a telescope that will tackle many different challenges in astronomy from observing the first galaxies that formed in the cosmos to revealing the processes that guide the birth of new stars.
But of all the potential discoveries that we might wonder about with this new telescope, it is the question of life elsewhere in the universe that most fiercely captures the public imagination.
So today, we will tackle the question that I know many of you are wondering.
Is JWST capable of detecting life beyond the solar system?
To begin this journey, we first need a primer on how it’s even possible to detect life from afar.
But if you wanna skip straight ahead to the results discussion, I’ll leave a timestamp down below for you.
First, it’s important to emphasize that we are really talking about any kind of life here, not necessarily intelligent.
After all, modern humans have only existed for about 1% of 1% of earth’s entire history.
The other 99.99% is dominated by the rest of the rich tapestry of living organisms.
Our search for life elsewhere in the universe largely focuses on bio-signatures, literally signatures of biology.
In other words, what sort of things might living systems do on a planetary scale that we might be able to detect from afar?
There’s many ways we could frame that, but most of the modern efforts revolve around the chemical composition of an exoplanet’s atmosphere because we’ve already demonstrated that we can detect molecules like water vapor, carbon dioxide, and ammonia.
So why not go a step further and detect the molecules indicative of life?
Now, when I say that, you might imagine I’m talking of molecules like DNA, RNA, or protein here, but our atmosphere contains negligible quantities of such complex molecules because they’re simply too heavy to be found at any useful altitude.
What we really need are abundant light molecules that life produces, something that wouldn’t be there if all life went extinct.
Fortunately, astrobiologists have come up with many suggestions as to what we might look for.
Arguably, the oldest proposed biosignature is oxygen, O2.
Something that Carl Sagan was advocating for back in 1993.
Plants, algae and cyanobacteria all engage in photosynthesis, which uses sunlight to combine water and carbon dioxide into oxygen and organic matter like glucose, for example.
It’s interesting to note that the oxygen is really a waste product here.
In fact, when photosynthesis first evolved on the earth, that oxygen was toxic, the then anaerobic life that dominated the biosphere.
That’s because molecular oxygen is highly reactive.
It doesn’t quite have enough electrons to fill its valence levels, which is energetically favorable, and so it’s hungry for electrons.
Hence, we call it an electron recipient or more simply, an oxidizer.
Now, it’s worth noting that photosynthesis alone isn’t enough to create a bio-signature.
To see this, imagine a plant that spends the summer photosynthesizing and producing, let’s say, X molecules of O2 that go into the air and a corresponding amount of molecules of organic matter that go into its structure.
Great, we have plenty of O2, but like everything else, that plant will eventually die, and then those organic molecules that it manufactured will decay.
During that process, bacteria are consuming the plant and respiring, which in total will require X molecules of oxygen to completely decay the organic matter.
And hence all the oxygen that that plant diligently produced ends up back where it started as CO2 and water.
So in order to get a surplus of oxygen, you need to somehow preserve that organic matter for it to get sequestered marine sediments and soils, for example.
That’s the only reason why the earth has a surplus of oxygen.
And what that means is that when we dig oil out of the ground and burn it, we’re interfering with that loop.
We are depleting the amount of oxygen in our atmosphere, but likewise increasing the amount of carbon dioxide.
Since about 2.4 billion years ago, a time known as the Great Oxidation Event, the Earth has been in oxygen surplus because of photosynthesis combined with organic sequestration.
So if we detected oxygen on another planet, it would be an incredible discovery then, indicating that something similar may be playing out there too.
So that’s it, right?
JWST just needs to detect O2 in an exoplanet atmosphere and we found life.
We’d have our Nobel Prize.
Unfortunately, it’s not quite that simple because it turns out that oxygen can be produced without biology, a process known as abiotic production.
A good example might be photolysis where ultraviolet radiation can split water up into H2 and O.
And for low mass planets like the earth, the H2 is actually light enough to escape into space, allowing oxygen to accumulate.
On earth, this admittedly only produces a tiny amount of oxygen, but it could plausibly be ramped up for stars with higher ultraviolet luminosities.
However, even at a slow drip drip rate of oxygen production that could accumulate over billions of years if the planet lacks any reducing agents.
Reducing agents are molecules that act as electron donors, chemicals that like to react with oxygen.
A good example might be methane.
In fact, methane plus oxygen is such a potent reaction that it’s one that SpaceX are mastering for their Raptor engines in their Starship project.
Now, if a planet lacks reducing agents though, oxygen could slowly build up and just linger around for a very long time.
For this reason, many have suggested that O2 isn’t really a bio-signature, at least not in isolation.
What you actually want is O2 plus a reducing agent.
That’s the combination, because those molecules should gradually react over time.
And so the fact you see lots of it hanging around implies that something is actively producing it, and that something here is life.
But another problem with O2 is that it’s damn hard to detect.
We detect molecules like this by looking at the spectra of an exoplanet.
That means we essentially look at the starlight that filters through the planetary atmosphere and we split it up into a rainbow using a prism.
Now, if you do this, you’ll notice that the spectrum of light is darker at certain specific wavelengths, indicative of a molecule absorbing that light at its characteristic vibrational or rotational frequency.
It turns out though, that O2, molecular oxygen, doesn’t really make much of a dent in the observable spectrum.
That’s unfortunate.
However, many have suggested that we could get ’round this by instead looking for ozone, O3, as a kind of proxy for molecular oxygen.
Ozone, O3, should really always exist whenever you have O2, as they constantly interchange between their two molecular states mediated by ultraviolet radiation in the upper atmosphere.
However, O3 is far easier to detect spectroscopically.
You might say that it has a far bigger footprint in the spectrum.
So that means that if we detect that O3 signature, then we know that O2 must be there by proxy even if we can’t detect it directly.
This is definitely very promising, but it does come with a downside.
And that is that using this technique, we probably won’t be able to tell exactly how much oxygen an exoplanet has.
That’s because the relationship between O2 and O3 is very non-linear, and it’s highly sensitive to the amount of ultraviolet radiance that that planet receives.
Nevertheless, the idea of detecting O3 plus a reducing agent like methane is a second example of a bio-signature that we could look for, and arguably a more promising one from a detection perspective.
Now, the role of oxygen is clearly pretty central to how life plays out on earth right now, but it hasn’t always been so.
Nor can we assume that other alien life will necessarily be the same as us.
The challenge then is to think outside of the box.
Besides oxygen, what other bio-signatures might occur?
Alien chemistry is surely very diverse.
I think we have to keep an open mind about that.
But at the same time, we can’t go around saying that every Tom, Dick or Harry molecule could be a product of life.
Otherwise every planet we observe would be plausibly keen to be alive.
It’s just not very satisfying or discerning to go around the universe pointing at every object and saying, “That might be alive, that might be alive.
” Instead, we would prefer to have a firm, compelling detection that minimizes plausible doubt.
So before I talk about bio-signature detectability with JWST, I’ll briefly highlight some other bio-signatures proposed by astrobiologists.
Probably the most popular one amongst my colleagues right now is nitrous oxide.
But you probably know it best as laughing gas.
And no, this isn’t popular because of its delirious effects on humans, but rather because of its potential as a detectable biosignature.
At least that’s the official stance.
Nitrous oxide is produced biotically on earth via incomplete denitrification of nitrate, NO3-2, molecular nitrogen N2.
This is a common product of anaerobic microbial organisms, and this could be the kind of a signature expected on earth before the Great Oxidation Event occurred.
Unfortunately, like oxygen, nitrous oxide can also be produced abiotically, such as through lightning, for example.
But again, it’s difficult to get high concentrations without a biosphere.
Besides nitrous oxide, other bio-signatures include certain sulfur gases like methyl mercaptan, which is produced by bacteria in wastelands, inland soils and coastal ecosystems.
Methane two is a classic biosignature, but it’s certainly not unambiguous, as Titan’s atmosphere will testify to.
Methyl chloride and bromide are more unusual suggestions that serve as a so-called capstone bio-signature.
So that’s one that’s harder to detect but could really solidify the case if it were found in combination with others.
And finally, phosphine has been proposed, and indeed there’s even hints for in the atmosphere of Venus, something that we’ve discussed on this channel before.
The claim, which has resurged interest in future space missions to our planetary cousin.
Like so many things in life then, you can see that this is a nuanced discussion, and it takes a bit of work to separate reality from mere rumor.
So there’s a few possible options for bio-signatures that we could go out and look for, but ultimately the question is, could JWST detect any of them?
Now, in any question of detectability, it is not just a question of the capabilities of the telescope itself, but also the target makes a huge difference.
For example, JWST can barely detect an entire galaxy if it’s on the other side of the universe, but it can trivially detect small asteroids orbiting around the solar system.
So clearly the target matters, and for now, it would seem that the best target is an exoplanet known as TRAPPIST-1e.
It’s a planet that ticks a lot of boxes.
It’s 91% the radius of the earth and 77% of the mass, but it orbits 34 times closer to its star than the Earth does.
Now, despite this, it actually receives less heat from its star than we do.
About 60% as much.
Why?
Because the host star TRAPPIST-1, is incredibly dim.
So dim in fact, that you can’t even see it with a naked eye despite the fact it’s just 40 light years away, which is relatively close on a cosmic scale.
TRAPPIST-1 is a so-called M dwarf star, a diminutive red dwarf star that’s so small, it’s in fact only barely big enough for hydro nuclear fusion to occur inside it at all.
And because it’s eight times smaller than our sun, that means that an earth size planet passing in front of it would block out eight squared, so 64 times more light than it would do if it were around our sun.
In fact, that’s precisely what makes TRAPPIST-1e so attractive for JWST.
The star itself provides a huge signal boost.
So to recap, the plan is then to observe the planet as it passes in front of the star, a so-called transit, take a spectrum of the atmosphere using JWST, and then see if we can detect any of the bio-signatures that we’ve covered thus far.
A detailed study led by Zifan Lin and colleagues from the Carl Sagan Institute, who by the way, have a great YouTube channel you can check out, tackled this question head on.
Simulating various atmospheric types and instrument modes, they report a somewhat pessimistic forecast for cracking open the champagne anytime soon.
If TRAPPIST-1e resembles modern earth, then they find that ozone could be detected to 2.4 sigma significance after collecting 200 transits of the planet in the optimal instrument configuration.
2.4 sigma just really isn’t much of a detection though, and frankly nobody’s gonna believe a claim at that level of significance.
Just to give some context, the Higgs boson was famously announced once it reached 5 sigma significance, and previously Kepler mandated that new exoplanetary transits had to be announced once they reached 7 sigma significance.
So let’s bump this up to say, 5 sigma, as a more convincing cutoff.
Using that and scaling Lin’s numbers, it turns out that you would need to accumulate 900 transits of TRAPPIST-1e using JWST.
900. That’s a lot.
Now it takes the planet 6.1 days to orbit its star.
That’s its year.
So every 6.1 days we get a chance to observe a transit.
The problem with that is that 900 multiplied by 6.1 days, equals 15 years, which considerably exceeds JWST’s primary mission lifetime of five years.
Now the mission will likely be extended beyond that, but eventually the spacecraft will simply run out of cryogen and rocket fuel, with current estimates capping the lifetime at about 10 years.
Accordingly, it’s sadly impossible for JWST to detect an earth-like ozone bio-signature at a level of significance that anyone’s likely to believe for the current best target.
Now, perhaps we’ll get lucky.
Perhaps TRAPPIST-1e will have way more ozone than the Earth does, or maybe a new target might emerge in the next few years that could supplant TRAPPIST-1e.
But hopefully this little demonstration proves to you that the idea of JWST detecting ozone is nontrivial.
It is really pushing credibility.
The best case scenario described in Lin’s paper is the agnostic combination of nitrous oxide and ozone.
So you are really not sure which one you’re detecting, but you know that one of them is there.
In that case, a 5 sigma detection could be achieved by accumulating 300 transits extrapolating off Lin’s numbers later.
Now 300 is still a huge number, but it seems at least possible in principle.
300 transits would correspond to five years of accumulated data, which is of course the lifetime of JWST.
But in practice it would actually be impossible to ever schedule that.
Because the problem is that JWST can’t physically point at the star all year round.
TRAPPIST-1 is unfortunately located in the constellation Aquarius near the ecliptic planes.
That’s the plane that JWST is itself orbiting around the sun.
And that means that for roughly half the year, TRAPPIST-1 is behind the sun from JWST’s perspective.
Needless to say, NASA are not gonna let you point a $10 billion telescope at the sun unless your goal is to annihilate all the instruments on board.
Accounting for the viewing constraints a bit more carefully, it turns out that TRAPPIST-1 is one is only visible for 100 days per year.
So each year we can only observe 16 transits at most of TRAPPIST-1e, and thus amassing 300 transits would take 19 years.
So again, way too long for us to seriously expect JWST to last that long.
In fact, I would go as far as to say that anything over about 80 transits is frankly unrealistic, especially because NASA are never going to agree to point JWST at this one target at every single opportunity, because after all, there are many other astronomers with competing and compelling science needs.
Despite this gloomy outlook, there is at least some interesting resource we can look forward to.
Lin and colleagues find that with 10 transits, carbon dioxide could be detected to 5 sigma, which might not be a bio-signature, but it would’ve at least established that the planet has an atmosphere of some kind.
An independent study led by Fauchez and colleagues repeated the calculation and they find that somewhere between 23 to 35 transits are necessary depending upon the CO2 levels assumed.
These studies make different assumptions about the treatment of clouds, hazes and other model details that lightly explain their discrepant answers.
But crucially, both broadly agree that JWST could at least detect the existence of an atmosphere for this enticing planet, which, let’s face it, would already be a major technical achievement.
The truth is, that if TRAPPIST-1e remains as our best target in the coming years, then a bio-signature detection looks to be a long way off.
And really that shouldn’t surprise us, because JWST was never actually designed to detect bio-signatures in the first place.
After all, we only knew of a handful of exoplanets when its design was first conceived.
As a longtime Tolkien fan, I am reminded of Gandalf’s words.
– There never was much hope.
– But remarkably, there is still a chance of success, but only if TRAPPIST-1e is usurped as the king of known earth-like exoplanets, for it’s possible that a new target could enter the fray, one that would change the game completely.
An earth-like planet orbiting a white dwarf.
Remember how I told you how TRAPPIST-1 reigned supreme because the star is both nearby and remarkably small, just 1/8 the size of the sun.
And that small size boosts our signal by a factor of 64.
But it is actually possible to have an even smaller star, a dead star.
After stars like the sun go giant, their cores are left behind and collapse into a dense ball of degenerate matter known as a white dwarf.
There’s no longer any fusion happening inside them, so they gradually diminish in brightness over billions of years.
But that process of cooling and slowly transforming into a so-called black dwarf takes so long, that if the white dwarf has a companion planet, that world could in principle retain a stable climate for billions of years.
And here’s the kicker, white dwarfs are so tiny, about 100 times smaller than the sun, in fact, that any exoplanetary transit signals would get boosted by a factor of a 100 squared, 10,000.
So that means the earth’s own white dwarfs would arguably present the absolute best case when it comes to detectability.
Now, sadly, we don’t have such a target right now.
We don’t know of any earth-sized planets which are orbiting around a white dwarf star at the right distance to be hospitable.
And frankly, for a long time, many have even doubted that such a thing is even possible.
After all, the orbit of that planet would have to be so close to the white dwarf that it would’ve once upon a time have been literally inside the star when that star was burning hydrogen.
So somehow you have to either move a survivor planet from far away to a much closer orbit, or perhaps even just form a whole new planet from the nebula of material leftover around the white dwarf.
It was easy to dismiss these scenarios as pretty unlikely until the discovery of the first transiting planet around a white dwarf in 2020, that’s WD 1856 b for short led by Andrew Vanderburg and colleagues.
Now, this isn’t an earth-like planet.
Rather it’s a Jupiter, but it at least proves that planets exist around these dead stars.
And furthermore, they can end up in very close in orbits.
So if, and admittedly it’s a big if, we discover a new transiting exoplanet around a similarly bright white dwarf as this, and if that planet happens to be fairly similar to the properties of the earth, then well, JWST would be rubbing his proverbial hands together in anticipation, because such a planet would be an astrobiologist’s dream come true.
Here, finally, JWST should be capable of delivering the goods home.
Another Sagan Institute study, this time led by their director and personal friend Lisa Kaltenegger, showed that bio-signatures would be evidently detectable here.
For example, both ozone and methane, the oxidizer and the reducing agent, could be detected to 5 sigma confidence within just 30 transits.
Or equally, one could detect ozone and nitrous oxide with similar numbers.
30 transit seems more reasonable, but let’s just check the schedule requirements quickly here.
Now, each one of these transits only lasts for about two minutes, but we’d still require about two hours on target in order to gather enough calibration data.
On top of that, let’s look at the orbital period.
Now, the temperate zone around white dwarfs is very, very close in, because those white dwarfs are so dim in the first place.
In fact, an earth-like planet around one of these stars would have an orbital period its year of just 10 hours.
The planet would to some degree be fighting against total disruption from the star itself with its survival dependent upon its exact viscosity parameters.
But assuming that it survives and is genuinely habitable, 30 transits here corresponds to just two weeks of continuous monitoring.
That’s the kind of program that I think we could realistically imagine NASA punting for.
Things might be even better for white dwarfs though.
But to see that, let’s take a step back.
Kaltenegger assumed that a transiting planet would be discovered around a white dwarf similar to the star WD 1856.
But the problem with this is that even if every single white dwarf has a planet like that, only 1% of them would have the right coincidental alignment to our line of sight that we could see them transit.
However, Mary Limbach led an innovative paper last year highlighting that for JWST, we need not limit ourselves to transiting planets though.
It could actually directly detect the light from the planet itself regardless as to whether it was transiting or not.
Essentially, the combined light of the white dwarf and the planet would be measured by JWST.
But because the planet is so much cooler than the white dwarf, its light would peak at an infrared wavelength separable from the star.
Here you really want an isolated white dwarf.
Binary systems don’t really work.
But within 21 light years, we know of six such white dwarfs.
Now, if one of those has an earth twin, the ozone plus methane bio-signature pair could be detected in just 36 hours of observational time to 5 sigma confidence with JWST.
The beauty here is that one need not wait for somebody to announce a new transiting planet around a white dwarf.
JWST could actually go ahead right now and survey the nearest white dwarfs for earth-like planets, taking about 10 hours of integration time per target.
In fact, I would confidently bet that Limbach put in a proposal just like that in the recent call for proposals that JWST had.
Do white dwarfs have earth size planets in the temperate zone?
Are those earths even habitable in the conventional sense?
Does life frequently spawn in such a distinct environment?
Those are all valid concerns, but at least we can actually observationally answer those questions.
So yes, it’s a long shot that white dwarfs have these earths.
It’s a long shot that the kind of stars we are focusing on here even have life to begin with.
And consequently, yes, it’s a long shot that JWST will detect bio-signatures.
I concede that.
But you know what else is true?
It’s possible.
Two words that have often kept me going through many a dark time in my life.
However outlandish your idea, your dream, whatever your peers may say about it, it’s possible.
And with JWST, it is eminently possible just about by maybe just a razor thin edge.
Yes, I admit that, but it’s possible that it could detect life in the next five, 10 years.
And if that’s not something to put a jazz in your step when you get get up in the morning, I don’t know what will.